Allosteric Interactive Drugs
Allosteric proteins regulate some of the most important biochemical pathways in organisms. Identification of allosteric binding site modulators have gained increased attention for their potential to be developed as selective therapeutic agents with fewer side effects. VCU medicinal chemists have a very long history of exploring the structure, function and biochemistry of hemoglobin, which is the most famous allosteric protein. Martin Safo’s group has continued this interest in understanding the structures, functions and regulatory mechanisms of the allosteric binding sites of hemoglobin, but has also studied pyruvate kinase, and 2,3-DPG mutase with respect to their potential as drug targets for a range of diseases. Using structure-based drug design, several hemoglobin allosteric effectors with ideal oxygen modulating properties potentially useful for the treatment of hypoxic diseases and sickle cell anemia have been discovered including 5-hydroxymethylfurfural (5-HMF), a safe, potent, and highly effective anti-sickling agent. 5-HMF was one of the first molecules to enter the NIH Therapeutics for Rare and Neglected Diseases program. The group of Umesh Desai is developing allosteric regulators of coagulation proteases such as thrombin, factor Xa, factor IXa, and factor XIa. Here, allosterism arises from cooperativity between the heparin-binding site and the active sites of these proteases. Small molecule glycosaminoglycan (GAG) mimetics that induce either full or partial inhibition of protease activity resulting in anticoagulation effects have been discovered. In vivo this translates to antithrombotic activity.
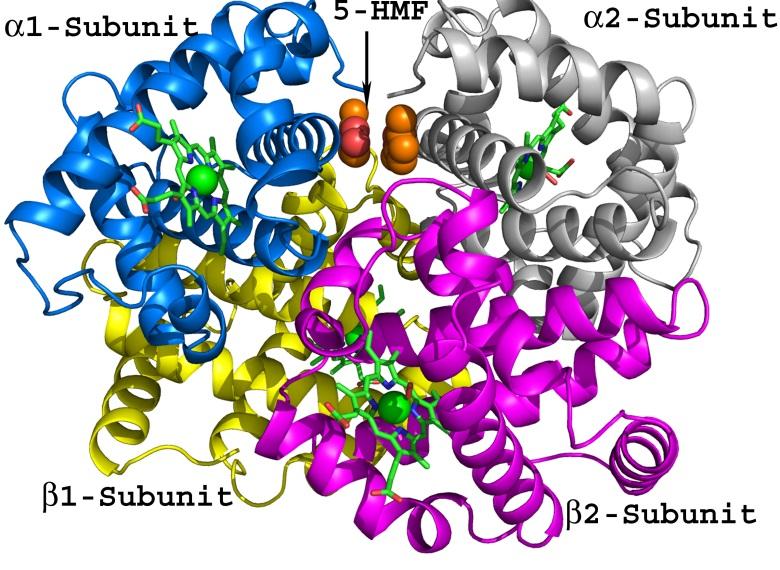